L23 Cellular motility
一、Muscle contraction
Myosins: actin-based motor proteins
Actin-Based Motor Proteins Are Members of the Myosin Superfamily
The first motor protein to be identified was skeletal muscle myosin, which generates the force for muscle contraction. This protein, now called myosin II, is an elongated protein formed from two heavy chains and two copies of each of two light chains
- Each heavy chain has a globular head domain at its N-terminus that contains the force-generating machinery, followed by a very long amino acid sequence that forms an extended coiled-coil that mediates heavy-chain dimerization
- Each myosin head binds and hydrolyzes ATP, using the energy of ATP hydrolysis to walk toward the plus end of an actin filament
A large family of motor proteins that can move along actin filaments, with ATP hydrolysis activity, > 40 members.
Myosins generate changes in cell shape and transport vesicles and organelles
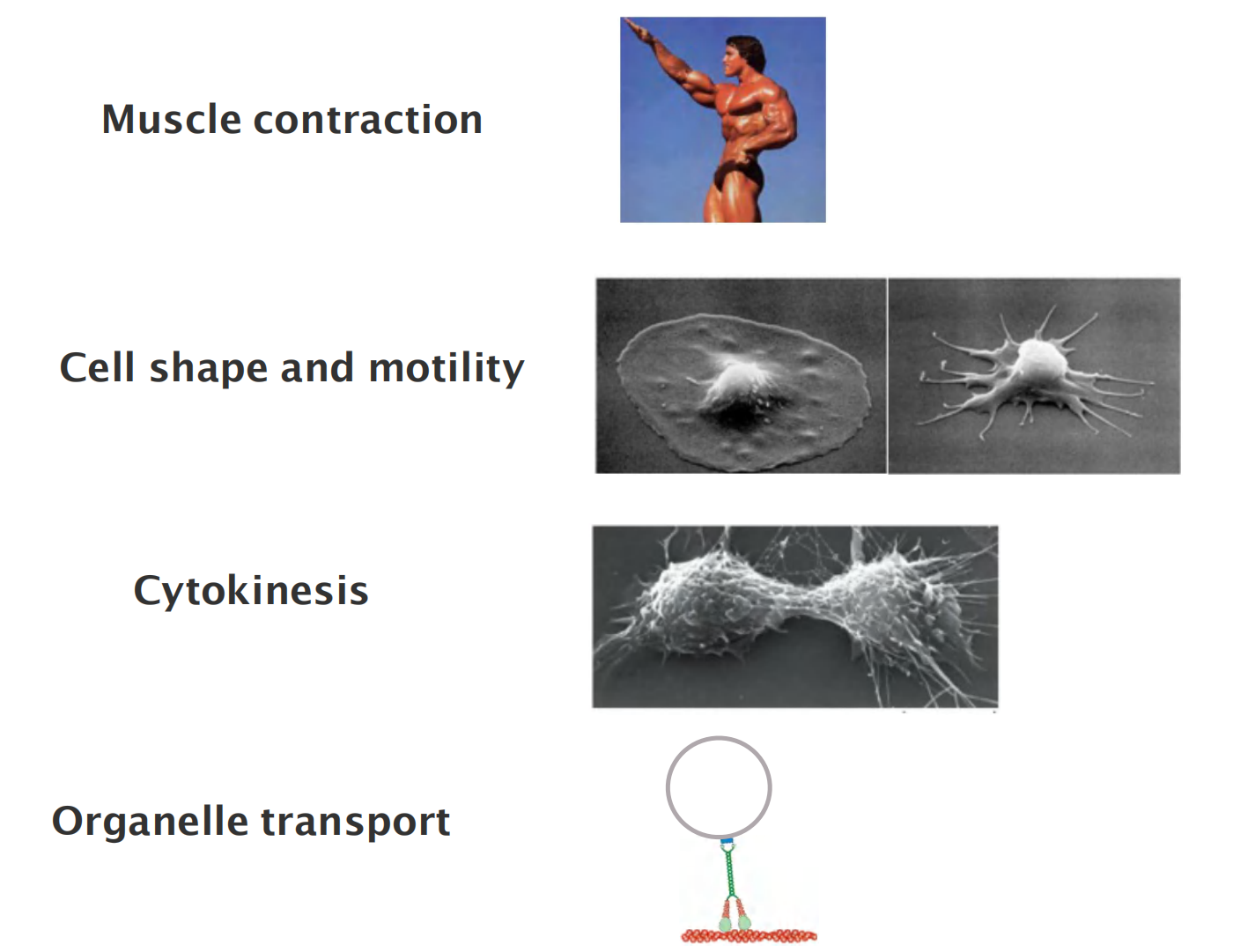
Muscle contraction
1. Structure of myosin II: muscle myosin
Two subfamilies of ATP-binding motor proteins
- Myosin-I have one head and one tail
- myosin-II (in muscle cells) are two-headed
- 2 heavy chains (abt. 2000 amino acids long)
- 2 essential light chains
- 2 regulatory light chains
- Head : S1 fragment , ATPase activity, actin binding sites
- Neck : light chains binding
- Tail: intertwining of two tail helices
2. Myosin II are arranged in a bipolar manner in skeletal muscle
The myosin II bipolar thick filament in muscles.
- (A) Electron micrograph of a myosin II thick filament isolated from frog muscle. Note: the central bare zone, which is free of head domains.
- (B) Schematic diagram, not drawn to scale. The myosin II molecules aggregate by means of their tail regions, with their heads projecting to the outside of the filament. The bare zone in the center of the filament consists entirely of myosin II tails.
3. Myosin head drives actin movement
In vitro motility assay
- purified myosin heads were attached to a glass slide.
- Phalloidin-labeled(鬼笔环肽标记) actin filaments were added and allowed to bind to the myosin heads.
- When ATP is added, the actin filaments began to glide along the surface, owing to the many individual steps taken by each of the dozens of myosin heads bound to each filament.
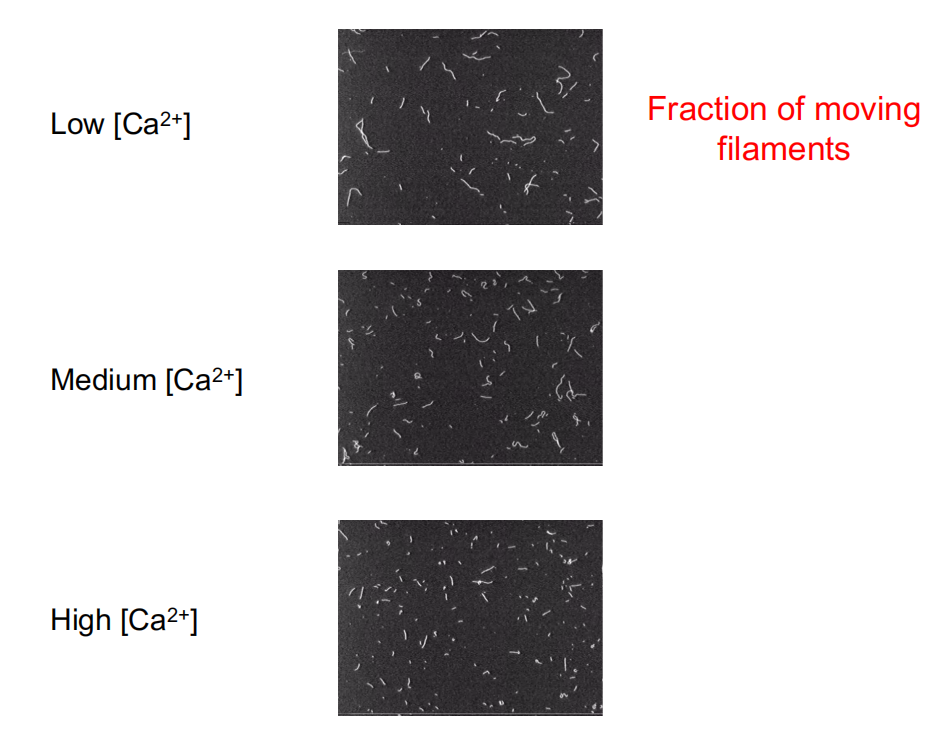
How a protein can walk in one direction?
An allosteric motor protein binds to ATP and changes different conformations includes a step driven by ATP hydrolysis of a bound ATP molecule, creating a “unidirectional ratchet” that makes the entire cycle essentially irreversible.
By repeated cycles, the protein therefore moves continuously to the right along uni-direction.
ATP hydrolysis couples myosin conformation change to cause movement along actin filaments
Myosin Generates Force by Coupling ATP Hydrolysis to Conformational Changes
For myosin II, each step of the movement along actin is generated by the swinging of an 8.5-nm-long α helix, or lever arm, which is structurally stabilized by the binding of light chains
These changes in the conformation of the myosin are coupled to changes in its binding affinity for actin
- allowing the myosin head to release its grip on the actin filament at one point and snatch hold of it again at another (并再次抢夺它)
The full mechanochemical cycle of nucleotide binding, nucleotide hydrolysis, and phosphate release (which causes the “power stroke”) produces a single step of movement
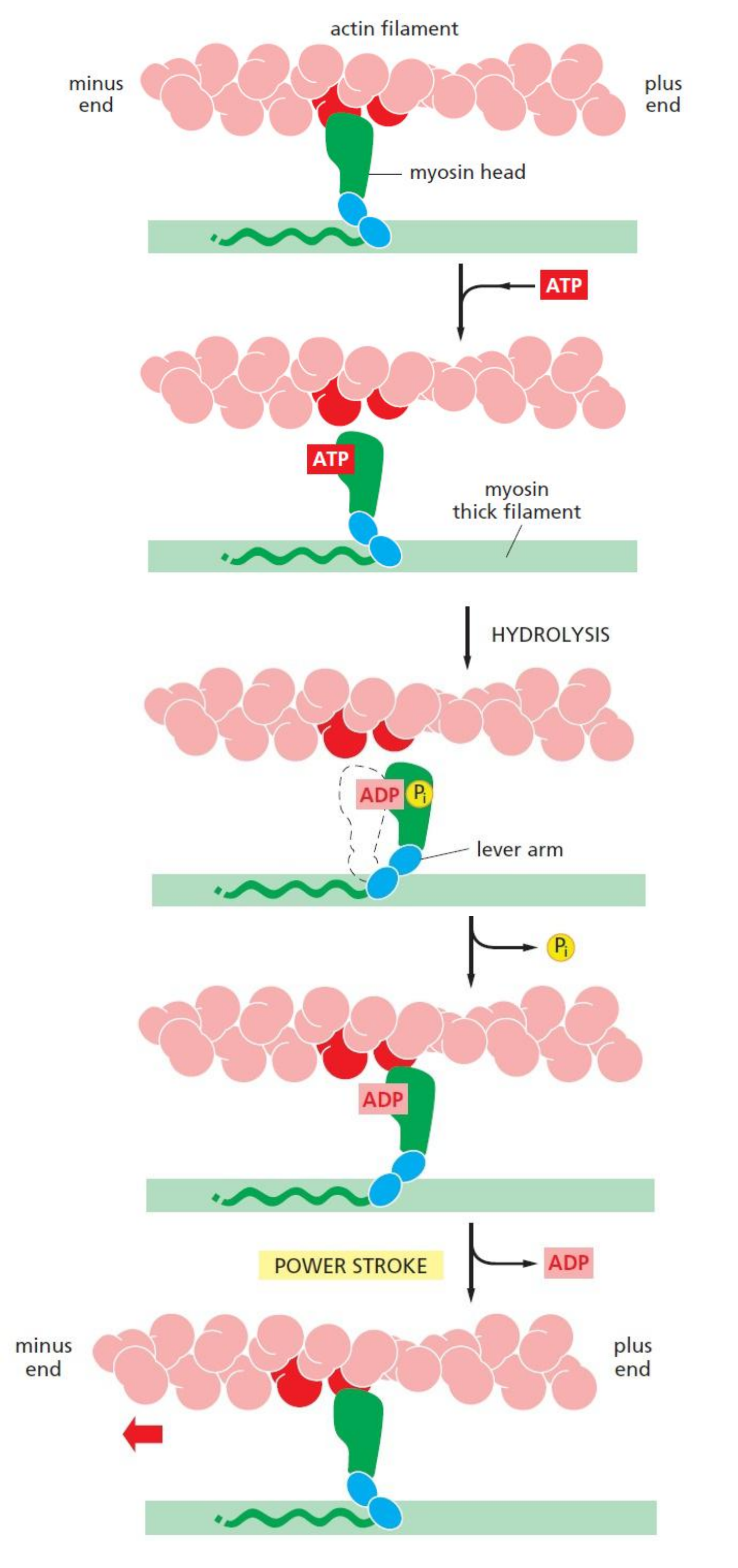
Sliding of Myosin II Along Actin Filaments Causes Muscles to Contract
In vertebrates, running, walking, swimming, and flying all depend on the rapid contraction of skeletal muscle on its scaffolding of bone, while involuntary movements such as heart pumping and gut peristalsis(蠕动) depend on the contraction of cardiac muscle and smooth muscle, respectively
Start: “Attached”:
- Myosin head is tightly locked to actin filament (Short-lived situation, terminated by ATP binding).
“Released”
- ATP-binding allows movement of the head along the actin filament.
“Cocked(竖起的)”
- Upon ATP binding, the cleft encloses the ATP, triggers movement of the lever arm, causing the displacement of the head along the filament (5 nm)
- After ATP hydrolysis, the inorganic phosphate (Pi) remains bound to the protein
“Force-generating”
- Weak binding of the head to a new actin site causes release of Pi, which triggers tight actin binding, causing the “power stroke”
- During the power stroke, the head loses the bound ADP, thus returning to the “attached” start position
Forces generation by myosin head measured by optical trap
At low ATP concentrations, the interval between the force-producing step and the binding of the next ATP is long enough that single steps can be observed
Muscle cells
The large muscle cell retains the many nuclei of the contributing cells. These nuclei lie just beneath the plasma membrane
- The bulk of the cytoplasm inside is made up of myofibrils(肌原纤维), which is the name given to the basic contractile elements of the muscle cell.
A myofibril is a cylindrical structure 1–2 μm in diameter that is often as long as the muscle cell itself.
- It consists of a long, repeated chain of tiny contractile units— called sarcomeres, each about 2.2 μm long—which give the vertebrate myofibril its striated(有沟痕的,有线条的,有条纹的) appearance
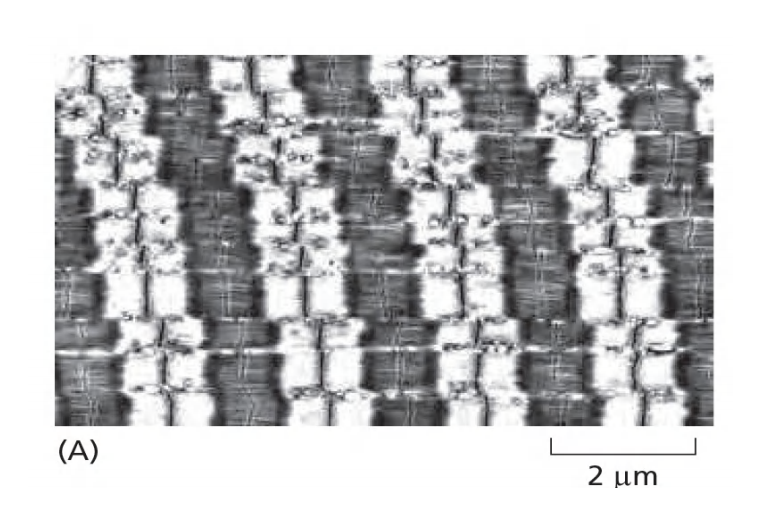
Myofibrils (1-2 µm in diameter) consist of sarcomeres (about 2 µm long), sarcomeres consist of actin and myosin II (contractile unit)
Myosin filaments start to pull actin filaments; this results in muscle contraction
These huge multinucleated cells form by the fusion of many muscle cell precursors, called myoblasts.
In an adult human, a muscle cell is typically 50 µm in diameter and can be up to several centimeters long.
1. Sarcomeres
Each sarcomere is formed from a miniature, precisely ordered array of parallel and partly overlapping thin and thick filaments
- The thin filaments are composed of actin and associated proteins, and they are attached at their plus ends to a Z disc at each end of the sarcomere
- The capped minus ends of the actin filaments extend in toward the middle of the sarcomere, where they overlap with thick filaments, the bipolar assemblies formed from specific muscle isoforms of myosin II
Cardiac muscle and smooth muscle also contain sarcomeres, although the organization is not as regular as that in skeletal muscle.
Sarcomere shortening is caused by the myosin filaments sliding past the actin thin filaments, with no change in the length of either type of filament
The rapid synchronized shortening of the thousands of sarcomeres lying end-to-end in each myofibril enables skeletal muscle to contract rapidly enough for running and flying, or for playing the piano.
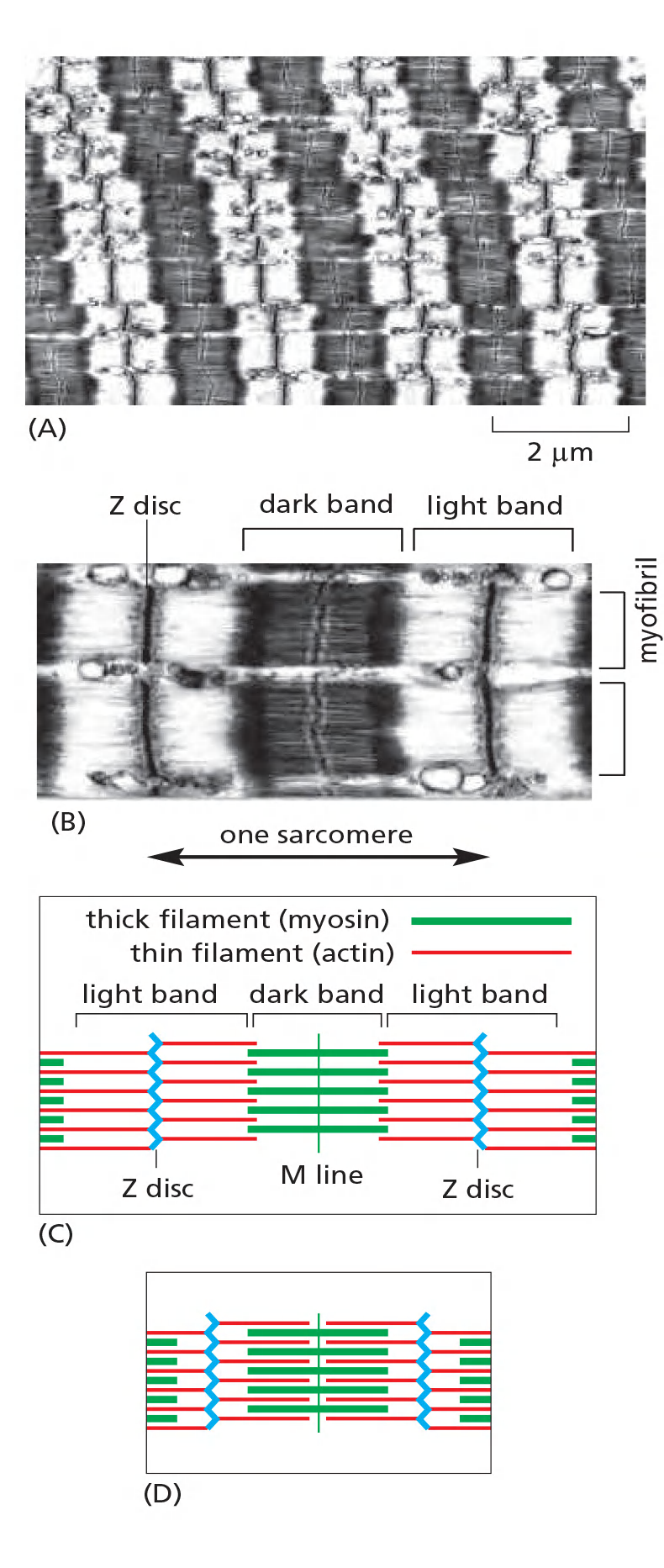
- The Z discs, at each end of the sarcomere, are attachment sites for the plus ends of actin filaments (thin filaments).
- The M line, or midline, is the location of proteins that link adjacent myosin II filaments (thick filaments) to one another
Contraction: actin and myosin filaments slide against each other without shortening
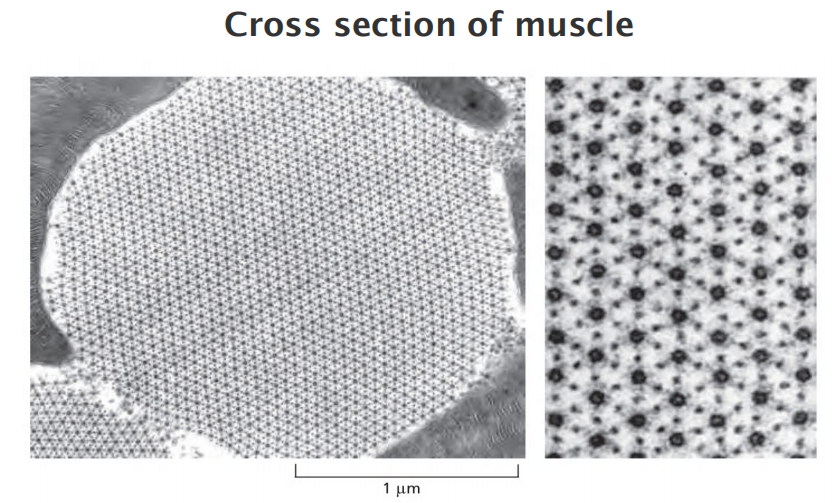
2. Organization of accessory proteins in a sarcomere
Accessory proteins produce the remarkable uniformity in filament organization, length, and spacing in the sarcomere
- The actin filament plus ends are anchored in the Z disc, which is built from CapZ and α-actinin;
- The precise length of each thin filament is influenced by a protein of enormous size, called nebulin(伴肌动蛋白), which consists almost entirely of a repeating 35-amino-acid actin-binding motif
- Nebulin stretches from the Z disc toward the minus end of each thin filament, which is capped and stabilized by tropomodulin(原调节蛋白)
Although there is some slow exchange of actin subunits at both ends of the muscle thin filament, such that the components of the thin filament turn over with a half-life of several days, the actin filaments in sarcomeres are remarkably stable compared with those found in most other cell types, whose dynamic actin filaments turn over with half-lives of a few minutes or less
- Nebulin: provide scaffold and structural support, molecular ruler
- Titin: a molecular spring
- CapZ and α-actinin: on the Z-line
- Tropomodulin: bind to minus end
Titin provides muscle with its elasticity
Opposing pairs of an even longer template protein, called titin, position the thick filaments midway between the Z discs
- Titin acts as a molecular spring, with a long series of immunoglobulin-like domains that can unfold one by one as stress is applied to the protein
- In C. elegans, whose sarcomeres are longer than those in vertebrates, titin is longer as well, suggesting that it serves also as a molecular ruler, determining in this case the overall length of each sarcomere.
T Tubules
T tubules (invagination from plasma membrane) relay the action potential (signal to contract) to the sarcoplasmic reticulum (SR), to all myofibrils of the cell (Lecture 8)
T tubules are extensions of the cell membrane that penetrate into the center of skeletal and cardiac muscle cells.
- The signal from the nerve triggers an action potential in the muscle cell plasma membrane, and spreads swiftly into T tubules.
- The signal is then relayed to the sarcoplasmic reticulum that surrounds each myofibril like a net stocking, and causes Ca2+ release channel to open transiently and release Ca2+ stored in the SR into the cytosol, which stimulate muscle contraction (for detailed myofibril contraction, see lecture 22).
Calcium
A Sudden Rise in Cytosolic Ca2+ Concentration Initiates Muscle Contractions
When the incoming action potential activates a Ca2+ channel in the T-tubule membrane, Ca2+ influx triggers the opening of Ca2+-release channels in the sarcoplasmic reticulum
- Ca2+ flooding into the cytosol then initiates the contraction of each myofibril.
The increase in Ca2+ concentration is transient because the Ca2+ is rapidly pumped back into the sarcoplasmic reticulum by an abundant, ATP-dependent Ca2+-pump (also called a Ca2+-ATPase) in its membrane
Thus, muscle contraction depends on two processes that consume enormous amounts of ATP: filament sliding, driven by the ATPase of the myosin motor domain, and Ca2+ pumping, driven by the Ca2+ pump.
Calcium triggers contraction of actin and myosin filaments
A sudden rise in cytosolic Ca2+ concentration initiates
muscle contraction
- Calcium signals are released in contacts between neural and muscle cells, and are going into muscle cells via T-tubules.
- In a places where T-tubules are close enough to ER (sarcoplasmatic reticulum), action potential “jumps” from T-tubule membrane to ER membrane and activate the Ca2+ rush from ER into cytosol.
- This is a contraction signal: calcium ions will interact with troponin-tropomyosin system which unblocks the myosin II
troponin and tropomyosin
One of these accessory proteins is a muscle form of tropomyosin(原肌球蛋白), the elongated protein that binds along the groove of the actin filament helix. The other is troponin(肌钙蛋白), a complex of three polypeptides, troponins T, I, and C (named for their tropomyosin-binding, inhibitory, and Ca2+-binding activities, respectively)
- Troponin I binds to actin as well as to troponin T.
- In a resting muscle, the troponin I–T complex pulls the tropomyosin out of its normal binding groove into a position along the actin filament that interferes with the binding of myosin heads, thereby preventing any force-generating interaction
- When the level of Ca2+ is raised, troponin C—which binds up to **four molecules of Ca2+**—causes troponin I to release its hold on actin. This allows the tropomyosin molecules to slip back into their normal position so that the myosin heads can walk along the actin filaments
Troponin C is closely related to the ubiquitous Ca2+-binding protein calmodulin (钙调蛋白)
- Troponin complex: T, I, and C
- (Tropomyosin-binding, Inhibitory, and Ca2+-binding activities)
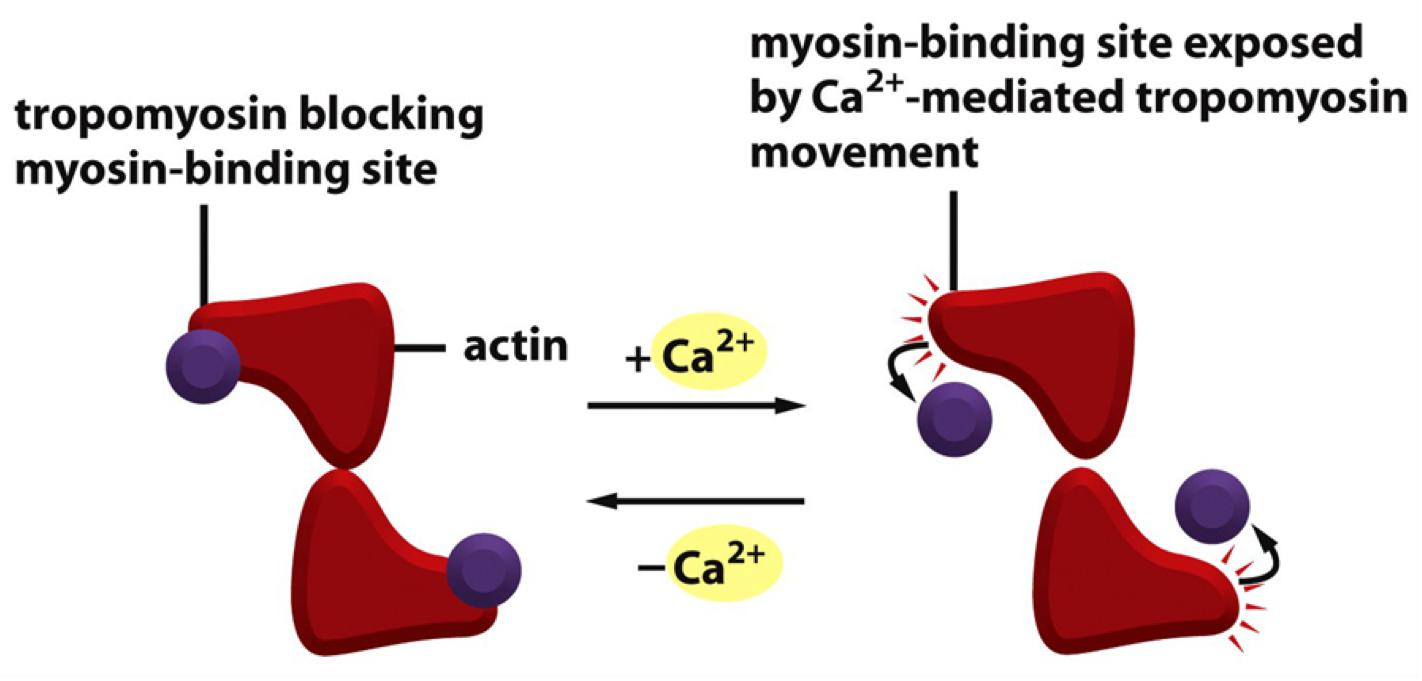
Resting muscle (-Ca2+):
- The troponin I–T complex pulls the tropomyosin out of its normal binding groove to block binding of myosin heads (no force generating action)
High Ca2+ :
- Troponin C causes troponin I to release its hold on actin.
- This allows the tropomyosin molecules to slip back into their normal position so that the myosin heads can walk along the actin filaments
Muscle contraction is additionally regulated by myosin II phosphorylation (e.g. smooth muscle contraction)
Smooth muscle forms the contractile portion of the stomach, intestine, and uterus, as well as the walls of arteries and many other structures requiring slow and sustained contractions.
Smooth muscle is composed of sheets of highly elongated spindle-shaped cells, each with a single nucleus.
- Smooth muscle cells do not express the troponins.
- Instead, elevated intracellular Ca2+ levels regulate contraction by a mechanism that depends on calmodulin
- Ca2+-bound calmodulin activates myosin light-chain kinase (MLCK), thereby inducing the phosphorylation of smooth muscle myosin on one of its two light chains.
- When the light chain is phosphorylated, the myosin head can interact with actin filaments and cause contraction; when it is dephosphorylated, the myosin head tends to dissociate from actin and becomes inactive.
Rapid activation of contraction is not important in smooth muscle: its myosin II hydrolyzes ATP about 10 times more slowly than skeletal muscle myosin, producing a slow cycle of myosin conformational changes that results in slow contraction
- Upon muscle stimulation by activation of cell-surface receptors, Ca2+ released into the cytoplasm from the sarcoplasmic reticulum (SR) binds to calmodulin.
- Ca2+-bound calmodulin then binds myosin light-chain kinase (MLCK), which phosphorylates myosin light chain, stimulating myosin activity.
Heart muscle contraction
Heart Muscle Is a Precisely Engineered Machine
Heart cells express several specific isoforms of cardiac muscle myosin and cardiac muscle actin.
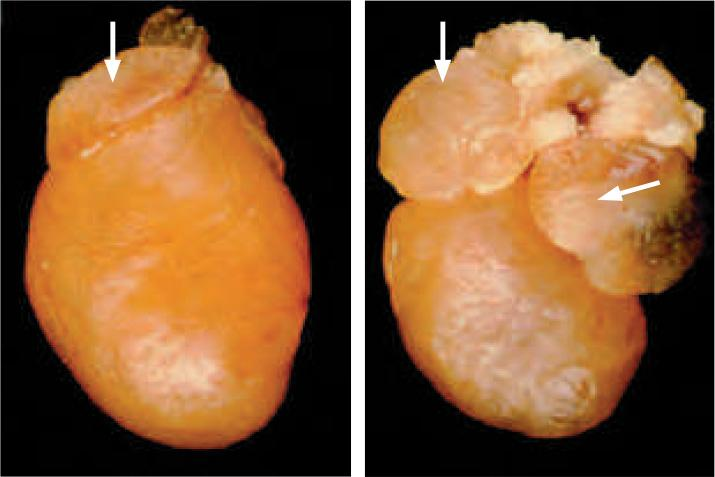
- Heart cells express several specific isoforms of cardiac muscle myosin and cardiac muscle actin.
- Even subtle changes in these cardiac-specific contractile proteins—changes that would not cause any noticeable consequences in other tissues—can cause serious heart disease: familial hypertrophic cardiomyopathy(家族性肥厚性心肌病), dilated cardiomyopathy(扩张型心肌病)
1. Actin and myosin in non-muscle cells
Actin and Myosin Perform a Variety of Functions in Non-Muscle Cells
Non-muscle contractile bundles are regulated by myosin phosphorylation rather than the troponin mechanism
These contractile bundles function to provide mechanical support to cells, for example, by assembling into cortical stress fibers that connect the cell to the extracellular matrix through focal adhesions or by forming a circumferential belt in an epithelial cell, connecting it to adjacent cells through adherens junctions (discussed in Chapter 19).
- As described in Chapter 17, actin and myosin II in the contractile ring generate the force for cytokinesis, the final stage in cell division.
All of the myosins except one move toward the plus end of an actin filament, although they do so at different speeds. The exception is myosin VI, which moves toward the minus end
myosin V moves continuously, or processively, along actin filaments without letting go
Myosin V motors carry a wide range of cargoes— including mRNA, endoplasmic reticulum, and secretory vesicles—along the actin cables and into the bud.
In addition, myosin V mediates the correct partitioning of organelles such as peroxisomes and mitochondria between mother and daughter cells
2 cytoskeletal isoforms in all non-muscle cells: β-, γ- actin
Non-muscle contractile bundles are regulated by myosin phosphorylation rather than the troponin mechanism.
These contractile bundles function to provide mechanical support to cells (Lecture 24, 25)
二、Whole-cell movement: cell crawling
Cell migration (crawling)
Many Cells Can Crawl Across a Solid Substratum
Cell migration is a complex process that depends on the actin-rich cortex beneath the plasma membrane. Three distinct activities are involved:
- protrusion(伸出,突出), in which the plasma membrane is pushed out at the front of the cell;
- attachment(附着, 附属), in which the actin cytoskeleton connects across the plasma membrane to the substratum;
- traction(牵引;牵引力), in which the bulk of the trailing cytoplasm is drawn forward
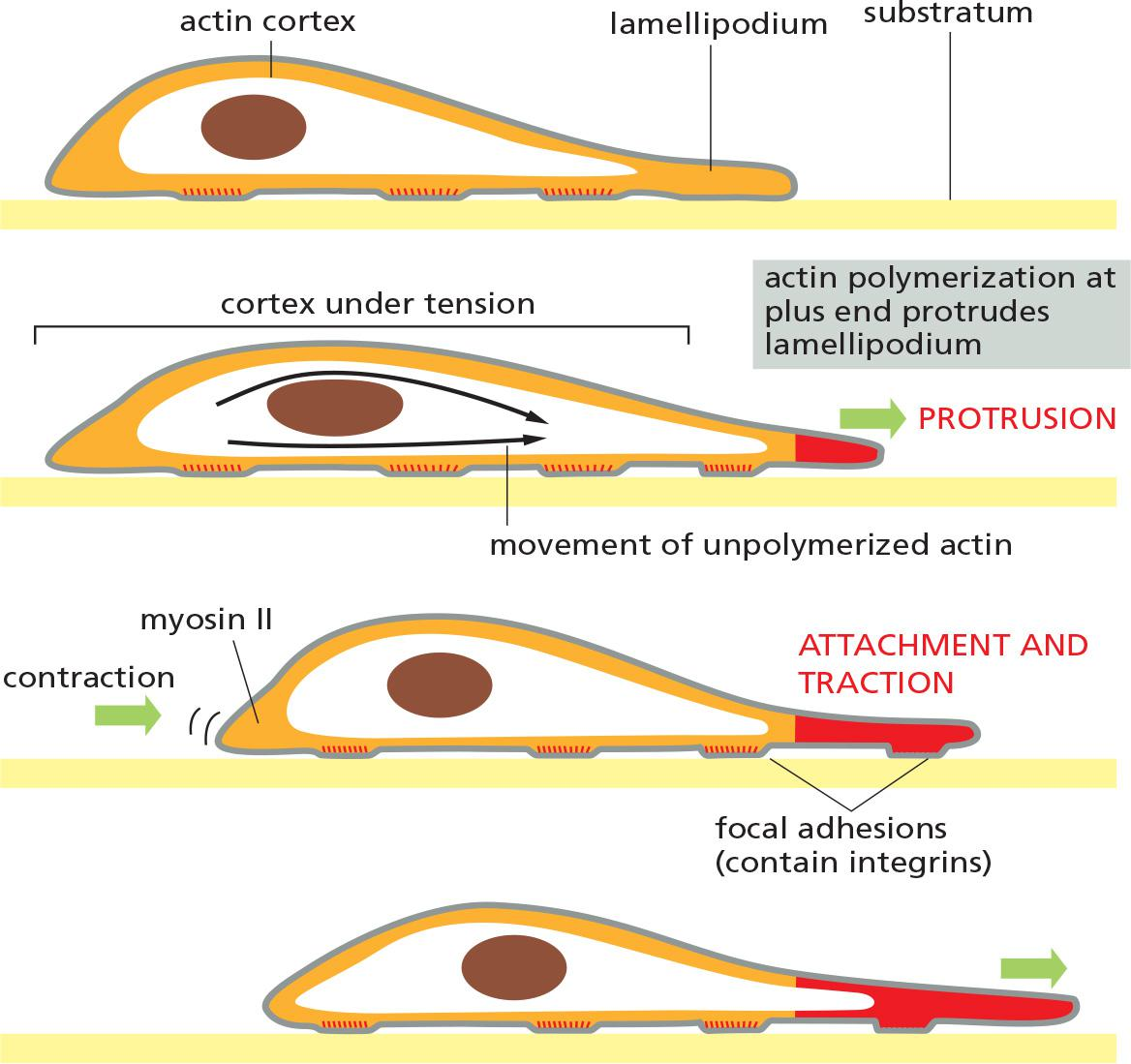
Focal adhesions, attachment
- Extension (Lamellipodium, Filopodia)
- New attachment
- (new focal adhesions)
- Cell contraction (rear retraction)
- Detachment
1. Protrusion and cell-substratum adhesion at the leading edge
Actin Polymerization Drives Plasma Membrane Protrusion
Different cell types generate different types of protrusive structures, including filopodia(丝状伪足) (also known as microspikes(微刺)) and lamellipodia(板状伪足). These are filled with dense cores of filamentous actin
- Actin polymerization at the cell front
- Actin retrograde flow in the absence of cell-substratum adhesion
- Protrusion at the leading edge in the presence of cell-substratum adhesion
Membrane blebbing
A distinct form of membrane protrusion called blebbing is often observed in vivo or when cells are cultured on a pliable extracellular matrix substratum.
Blebs form when the plasma membrane detaches locally from the underlying actin cortex, thereby allowing cytoplasmic flow to push the membrane outward
Bleb formation also depends on hydrostatic pressure(静水压力) within the cell, which is generated by the contraction of actin and myosin assemblies. Once blebs have extended, actin filaments reassemble on the bleb membrane to form a new actin cortex
Recruitment of myosin II and contraction of actin and myosin can then power retraction of membrane blebs. Alternatively, extension of new blebs from old ones can drive cell migration.
Membrane blebbing
- the plasma membrane detaches locally from the underlying actin cortex
- cytoplasmic flow to push the membrane outward
- Bleb formation depends on hydrostatic pressure within the cell, which is generated by the contraction of actin and myosin assemblies.
- Once blebs have extended, actin filaments reassemble on the bleb membrane to form a new actin cortex.
1. Migratory fish epithelial keratocytes
Simple reproducible cell shapes and cytoskeletal structures enable integrated experimental and theoretical approaches
Comprehensive whole-cell models incorporating details of molecular interaction with large-scale force balance and protein distribution and transport
2. Actin filament nucleation and web formation in lamellipodia
Filopodia(丝状伪足), formed by migrating growth cones of neurons and some types of fibroblasts, are essentially one-dimensional. They contain a core of long, bundled actin filaments, which are reminiscent of those in microvilli but longer and thinner, as well as more dynamic.
Lamellipodia(板状伪足), formed by epithelial cells and fibroblasts, as well as by some neurons, are two-dimensional, sheetlike structures. They contain a cross-linked mesh of actin filaments, most of which lie in a plane parallel to the solid substratum.
Invadopodia(侵袭性伪足) and related structures known as podosomes(足小体) represent a third type of actin-rich protrusion. These extend in three dimensions and are important for cells to cross tissue barriers, as when a metastatic cancer cell invades the surrounding tissue.
- Invadopodia contain many of the same actin-regulatory components as filopodia and lamellipodia, and they also degrade the extracellular matrix, which requires the delivery of vesicles containing matrix-degrading proteases.
Lamellipodia Contain All of the Machinery Required for Cell Motility
The actin filaments in the meshwork are mostly oriented with their plus ends facing forward. The minus ends are frequently attached to the sides of other actin filaments by Arp 2/3 complexes
The web as a whole is undergoing treadmilling, assembling at the front and disassembling at the back
Formation of lamellipodia
Myosin Contraction and Cell Adhesion Allow Cells to Pull Themselves Forward
Myosin II operates in at least two ways to assist cell migration.
Forces generated by both actin polymerization and myosin activity create tension at attachment sites, promoting their maturation into focal adhesions
Actin-mediated protrusions can only push the leading edge of the cell forward if there are strong interactions between the actin network and the focal adhesions that link the cell to the substrate.
- When these interactions are disengaged, polymerization pressure at the leading edge and myosin-dependent contraction cause the actin network to slip back, resulting in a phenomenon known as retrograde flow
Web of growing actin filament will push the leading edge of pseudopodium forward
Arps are starting points of new filaments, formins promote filament growing
Delayed ATP hydrolysis provides mechanisms of efficient and unidirectional treadmilling process in lamellipodia
Involves the actin binding protein cofilin
Rho family small GTPases regulate cell polarity and migration
Cell Polarization Is Controlled by Members of the Rho Protein Family
In many cases, including but not limited to cell migration, large-scale cytoskeletal coordination takes the form of the establishment of cell polarity, where a cell builds different structures with distinct molecular components at the fronts versus the back, or at the top versus the bottom.
The establishment of many kinds of cell polarity depends on the local regulation of the actin cytoskeleton by external signals
Many of these signals seem to converge inside the cell on a group of closely related monomeric GTPases that are members of the Rho protein family—**Cdc42**, Rac, and Rho
- Activation of Cdc42 on the inner surface of the plasma membrane triggers actin polymerization and bundling to form filopodia
- Activation of Rac promotes actin polymerization at the cell periphery, leading to the formation of sheetlike lamellipodial extensions
- Activation of Rho promotes both the bundling of actin filaments with myosin II filaments into stress fibers and the clustering of integrins and associated proteins to form focal adhesions
Some key targets of activated Cdc42 are members of the WASp protein family
- WASp proteins can exist in an inactive folded conformation and an activated open conformation. Association with Cdc42-GTP stabilizes the open form of WASp, enabling it to bind to the Arp 2/3 complex and strongly enhance its actin-nucleating activity
Rac-GTP also activates WASp family members.
- Additionally, it activates the cross-linking activity of the gel-forming protein **filamin**(肌丝蛋白) and inhibits the contractile activity of the motor protein myosin II.
- It thereby stabilizes lamellipodia and inhibits the formation of contractile stress fibers
Rho-GTP has a very different set of targets. Instead of activating the Arp 2/3 complex to build actin networks, Rho-GTP turns on formin proteins to construct parallel actin bundles
- At the same time, Rho-GTP activates a protein kinase that indirectly inhibits the activity of cofilin, leading to actin filament stabilization.
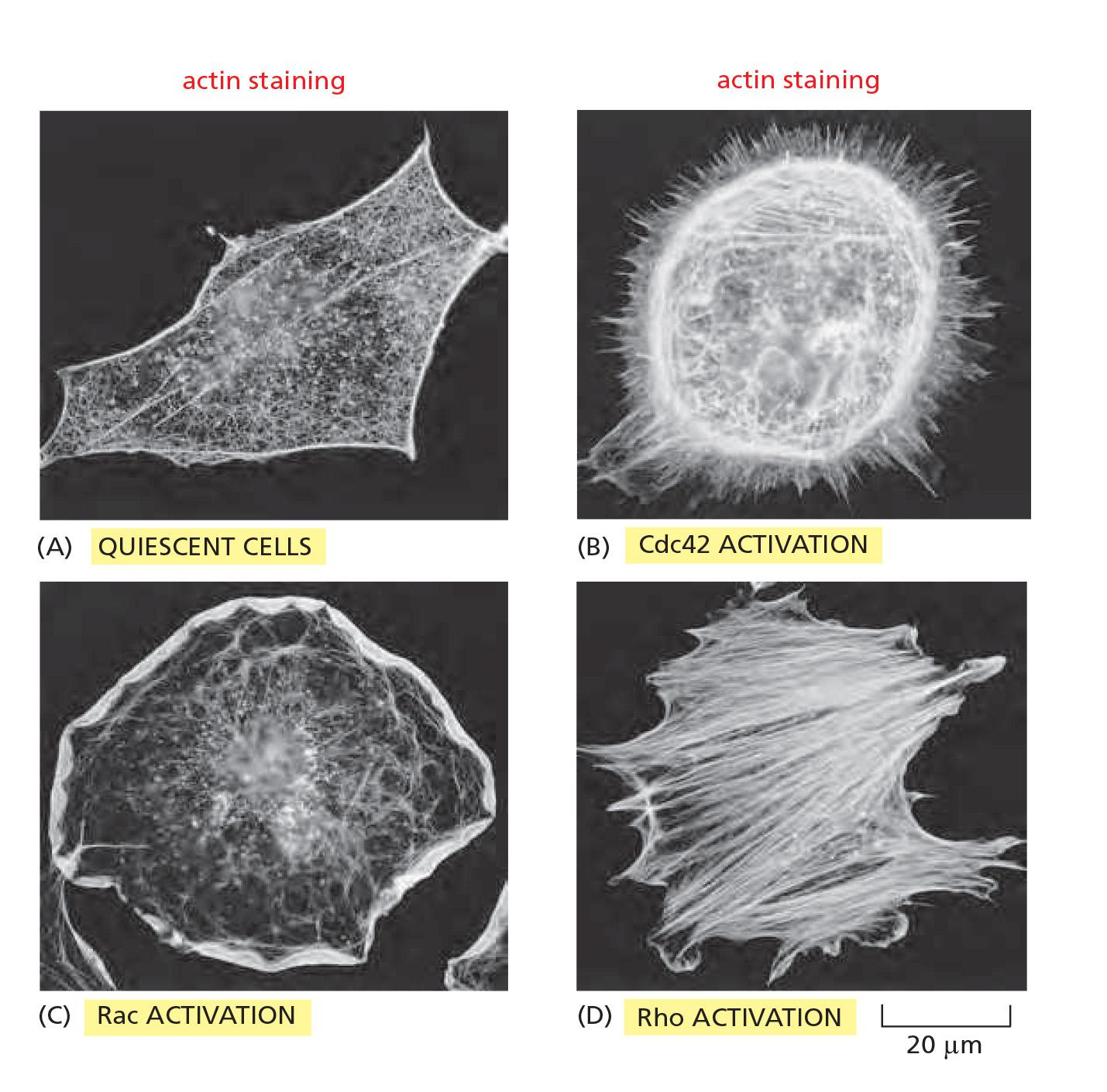
Neutrophil in chemotaxis (趋化作用,趋化现象,趋药性)
TWO distinct signaling pathways contribute to the cell’s polarization.
- Binding of bacterial molecules to G-protein-coupled receptors on the neutrophil stimulates directed motility.
- At the front of the cell: stimulation of the Rac pathway via the trimeric G-protein Gi, triggers growth of protrusive actin networks in the front.
- Second messengers within this pathway are short-lived, so protrusion is limited to the region of the cell closest to the stimulant.
- The same receptor also stimulates a second signaling pathway, via the trimeric G proteins G12/13, which triggers Rho activation.
- The two pathways are mutually antagonistic (敌对的;对抗性的).
- Rac-based protrusion is active at the front of the cell, Rho is activated only at the rear of the cell, stimulating contraction of the cell rear and assisting directed movement.
1. Coordinates whole-cell polarization and locomotion through communication among cytoskeletal elements
Cell migration is driven primarily by actin polymerization and myosin contractility, but septins and intermediate filaments (vimentin) also participate.
Disruption of linker proteins (plaskins) that connect different cytoskeletal elements, including several plakins and KASH proteins leads to defects in cell polarization and migration.
Cells also use microtubules to help organize persistent movement in a specific direction.
Several effector proteins downstream of Rac and Rho modulate microtubule dynamics directly.
Microtubule influence actin rearrangement and cell adhesion.
三、Microtubule motor proteins
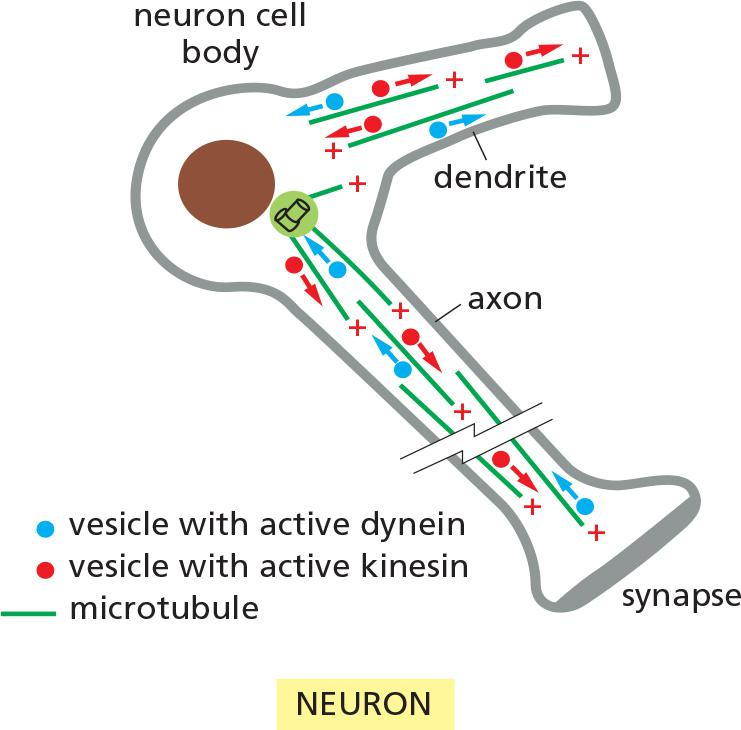
- Both axons and dendrites are filled with microtubule bundles that are critical to both their structure and their function.
- In axons, all the microtubules are long and oriented in the same direction, with their minus end pointing back toward the cell body and their plus end pointing toward the axon terminals.
- Dendrites contain much shorter microtubule with mixed polarities
Studying microtubule
Isolate axon from giant squid, ~1 mm in diameter:
- Cut axons into segments
Cell free system:
- ATP (AMP-PNP)
- taxol-stabilized microtubule
- purified organelle (labelled)
- axon extract (organelle free)
Findings:
- Transport occurs bi-directionally (anterograde and retrograde).
- Some are transported fast, some slow.
- By providing AMP-PNP, motor proteins bindto microtubule and organelles tightly without dissociation.
- Identify kinesin 1 and other MAPs
Microtubule motor proteins
- Kinesins (anterograde/forward) and dyneins (retrograde/backward) are dimers that hydrolyze ATP and move They can move molecules and even whole organelles
1. Kinesins
Two Types of Motor Proteins Move Along Microtubules
There are two major classes of microtubule-based motors, kinesins and dyneins.
- Kinesin-1, also called “conventional kinesin,” was first purified from squid neurons, where it carries membrane-enclosed organelles away from the cell body toward the axon terminal by walking toward the plus end of microtubules.
- Kinesin-1 is similar to myosin II in having two heavy chains per active motor; these form two globular head motor domains that are held together by an elongated coiled-coil tail that is responsible for heavy-chain dimerization.
Some kinesin heavy chains are homodimers, and others are heterodimers.
- Kinesins family proteins for anterograde movement (toward plus end)
Diverse functions:
- organelle transport,
- mRNA transport,
- chromosome transport
- microtubule sliding
- microtubule depolymerization
Mechanistic understanding of kinesin function
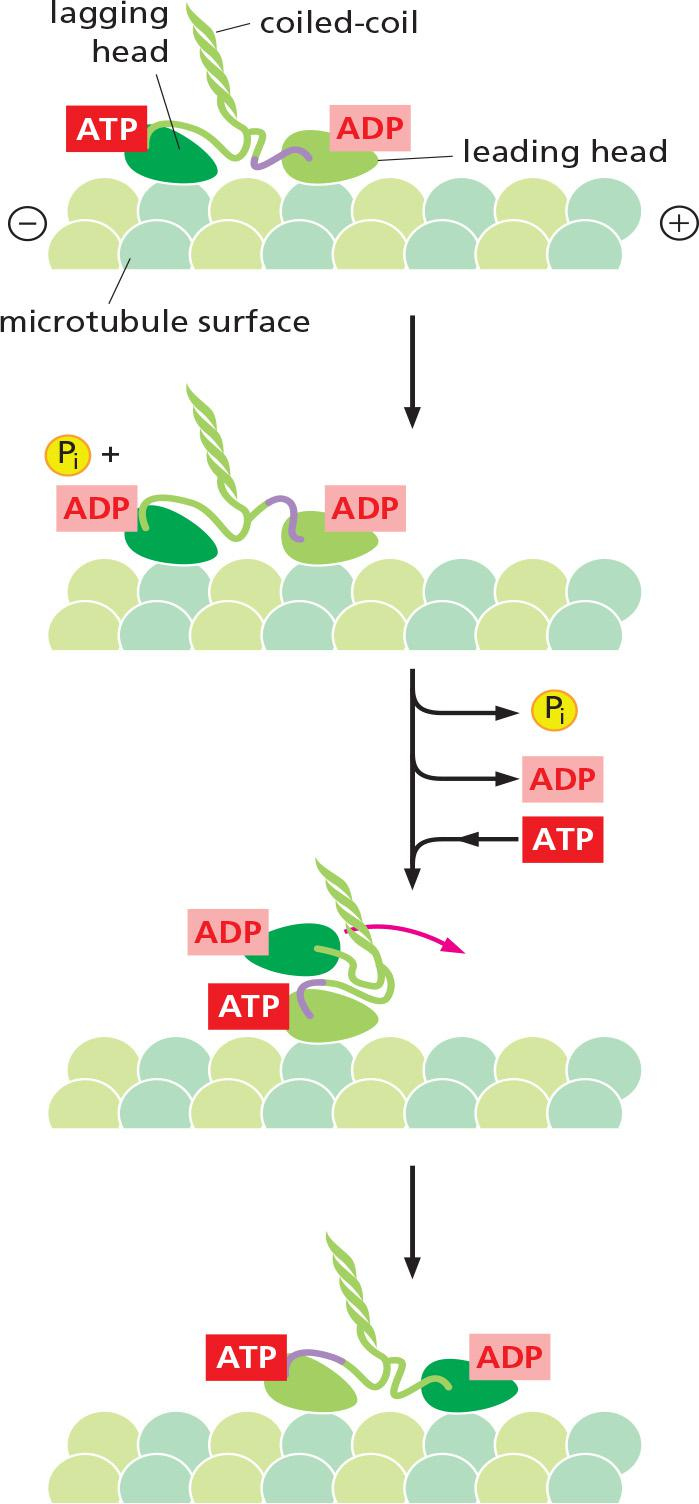
- Always associate with microtubules
- “ hand-over-hand” motion
- ADP-kinesin binds weakly to tubule
- ATP-kinesin binds strongly to tubule
Forward displacement of rear motor domain (lagging head) is driven by dissociation of ADP and binding of ATP in the leading head.
ATP binding causes a small peptide called the “neck linker” to shift from a rearward-pointing to a forward-pointing conformation.
This shift pulls the rear head forward. Detachment requires ATP hydrolysis and phosphate (Pi) release. Once it has detached from the microtubule, it binds to ADP.
The kinesin molecule is now poised for the next step, which proceeds by an exact repeat of the process shown.
2. Dynein
The dyneins are a family of minus-end directed microtubule motors unrelated to the kinesins. They are composed of one, two, or three heavy chains (that include the motor domain) and a large and variable number of associated intermediate, light-intermediate, and light chains
The dynein family has two major branches
- The first branch contains the cytoplasmic dyneins, which are homodimers of two heavy chains
- Cytoplasmic dynein 1 is used for organelle and mRNA trafficking, for positioning the centrosome and nucleus during cell migration, and for construction of the microtubule spindle in mitosis and meiosis
- Cytoplasmic dynein 2 is found only in eukaryotic organisms that have cilia and is used to transport material from the tip to the base of the cilia, a process called intraflagellar transport
- Axonemal dyneins (also called ciliary dyneins(睫状动力蛋白)) comprise the second branch and include monomers, heterodimers, and heterotrimers, with one, two, or three motor-containing heavy chains, respectively
- They are highly specialized for the rapid and efficient sliding movements of microtubules that drive the beating of cilia and flagella
Dyneins are the largest of the known molecular motors, and they are also among the fastest
Dynein family proteins for retrograde movement (toward minus end)
It is a very large multi-subunit protein.
They are composed of one, two, or three heavy chains (that include the motor domain) and a large and variable number of associated intermediate, light-intermediate, and light chains.
Two categories>: cytoplasmic dynein and axonemal dynein (e.g. ciliary dynein)
Mechanistic understanding of dynein function
- Each power stroke generates a step of about ~ 8 nm towards its minus end.
Dynein and dynactin
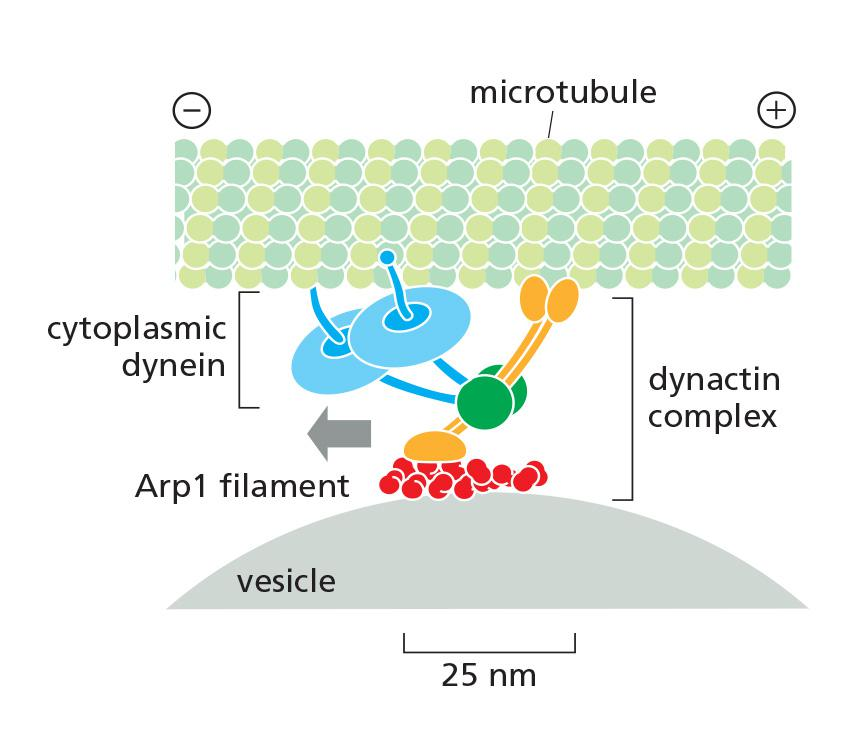
It itself can’t transport cargo directly, and needs dynactin to link to cargo to be transported
Microtubules and Motors Move Organelles and Vesicles
Kinesin was originally identified as the protein responsible for fast anterograde axonal transport
Cytoplasmic dynein was identified as the motor responsible for transport in the opposite direction, retrograde axonal transport.
Centripetal movements of organelles or vesicles toward the cell center require the action of minus-end directed cytoplasmic dynein motors, whereas centrifugal movements toward the periphery require plus-end directed kinesin motors.
The network of ER membrane tubules aligns with microtubules and extends almost to the edge of the cell
The different tails and their associated light chains on specific motor proteins allow the motors to attach to their appropriate organelle cargo.
Cytoplasmic dynein, itself a huge protein complex, requires association with a second large protein complex called dynactin to translocate organelles effectively.
- The dynactin complex includes a short, actin-like filament that forms from the actin-related protein Arp1 (distinct from Arp2 and Arp3, the components of the Arp 2/3 complex involved in the nucleation of conventional actin filaments)
Dynein is required continuously for neuronal function, as mutations in a dynactin subunit or in the tail region of cytoplasmic dynein lead to neuronal degeneration in humans and mice.
四、Flagella and cilia
Hair-like structures growing from cytoplasmic basal bodies
Contain 9X2 + 2 microtubules connected by dynein arms They are natural oars
9 doublet microtubules (1 of the 2 is complete) are arranged around a pair (two) of single microtubules (9+2 configuration)
Basal bodies: microtubule assembly sites for cilia and flagella
Construction of Complex Microtubule Assemblies Requires Microtubule Dynamics and Motor Proteins
- Spindle assembly depends on the coordinated actions of several motor proteins and other factors that modulate polymerization dynamics
Both axons and dendrites (collectively called neurites) are filled with bundles of microtubules that are critical to both their structure and their function.
Motile Cilia and Flagella Are Built from Microtubules and Dyneins
- Both cilia and flagella are hairlike cell appendages that have a bundle of microtubules at their core.
- Flagella are found on sperm and many protozoa.
Cilia are organized in a similar fashion, but they beat with a whiplike motion (鞭状运动)
In the human body, huge numbers of cilia (109/cm2 or more) line our respiratory tract, sweeping layers of mucus, trapped particles of dust, and bacteria up to the mouth where they are swallowed and ultimately eliminated
- Likewise, cilia along the oviduct help to sweep eggs toward the uterus.
The movement of a cilium or a flagellum is produced by the bending of its core, which is called the axoneme. The axoneme is composed of microtubules and their associated proteins, arranged in a distinctive and regular pattern
- accessory proteins cross-link the microtubules together.
Molecules of axonemal dynein form bridges between the neighboring doublet microtubules around the circumference of the axoneme
However, the presence of other links between the microtubule doublets prevents this sliding, and the dynein force is instead converted into a bending motion
- Firmly root eukaryotic cilia and flagella at the cell surface.
- Similar to centrioles
EM of a section through 3 basal bodies:
9 groups of fused triplet microtubules in a cartwheel configuration. 1 of the 3 fused microtubules is complete, that is the A microtubule
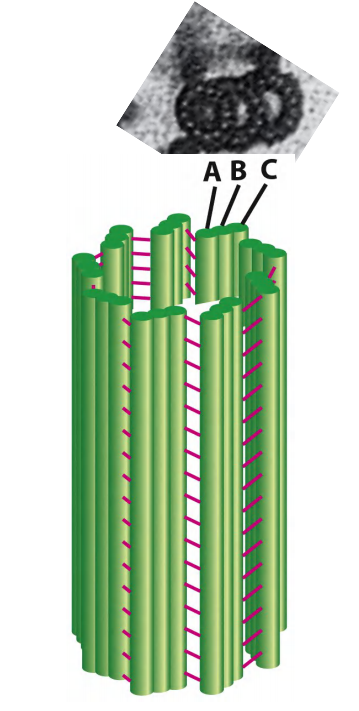
Ciliary dynein
- Cilia dynein is a large protein assembly (nearly 2 million daltons) composed of 9–12 polypeptide chains, the largest of which is the heavy chain of more than 500,000 daltons.
- The heavy chains form the major portion of the globular head and stem domains, and many of the smaller chains are clustered around the base of the stem.
- There are two heads in the outer dynein in metazoans but three heads in protozoa, each formed from their own heavy chain.
- The tail of the molecule binds tightly to microtubule.
Cilia and flagella: similar components, different action
bacterial flagella are long, rigid helical filaments, made up of repeating subunits of the protein flagellin. The flagella rotate like propellers, driven by a special rotary motor embedded in the bacterial cell wall.
Primary Cilia Perform Important Signaling Functions in Animal Cells
Many cells possess a shorter, nonmotile counterpart of cilia and flagella called the primary cilium
Both motile and nonmotile cilia are generated during interphase at plasma-membrane-associated structures called basal bodies that firmly root them at the cell surfaces
Because no protein translation occurs in cilia, construction of the axoneme requires intraflagellar transport (IFT),
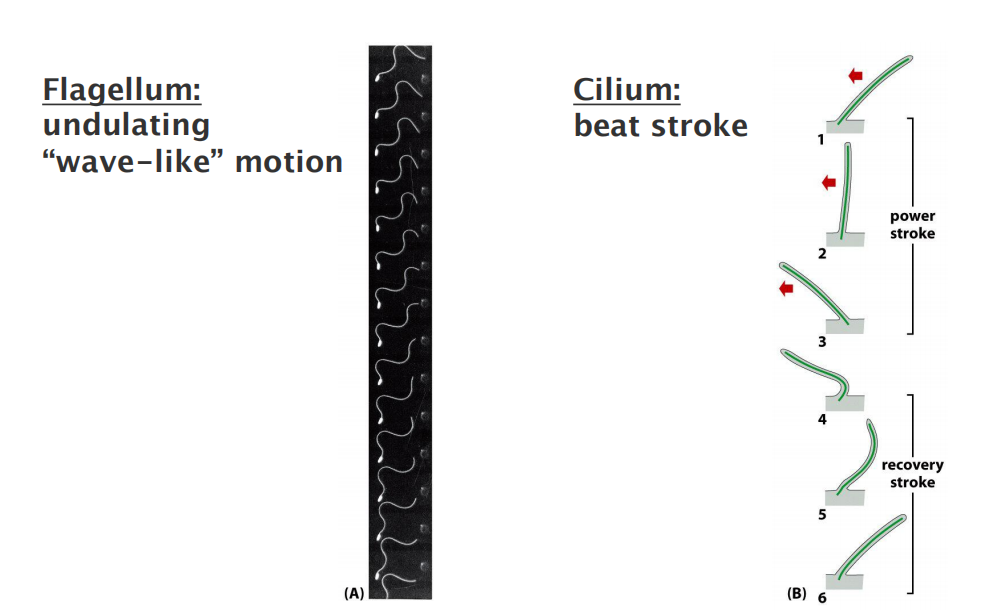
- Cilia and flagella are both built from microtubules and dynein but have totally different modes of motion
Flagella bending
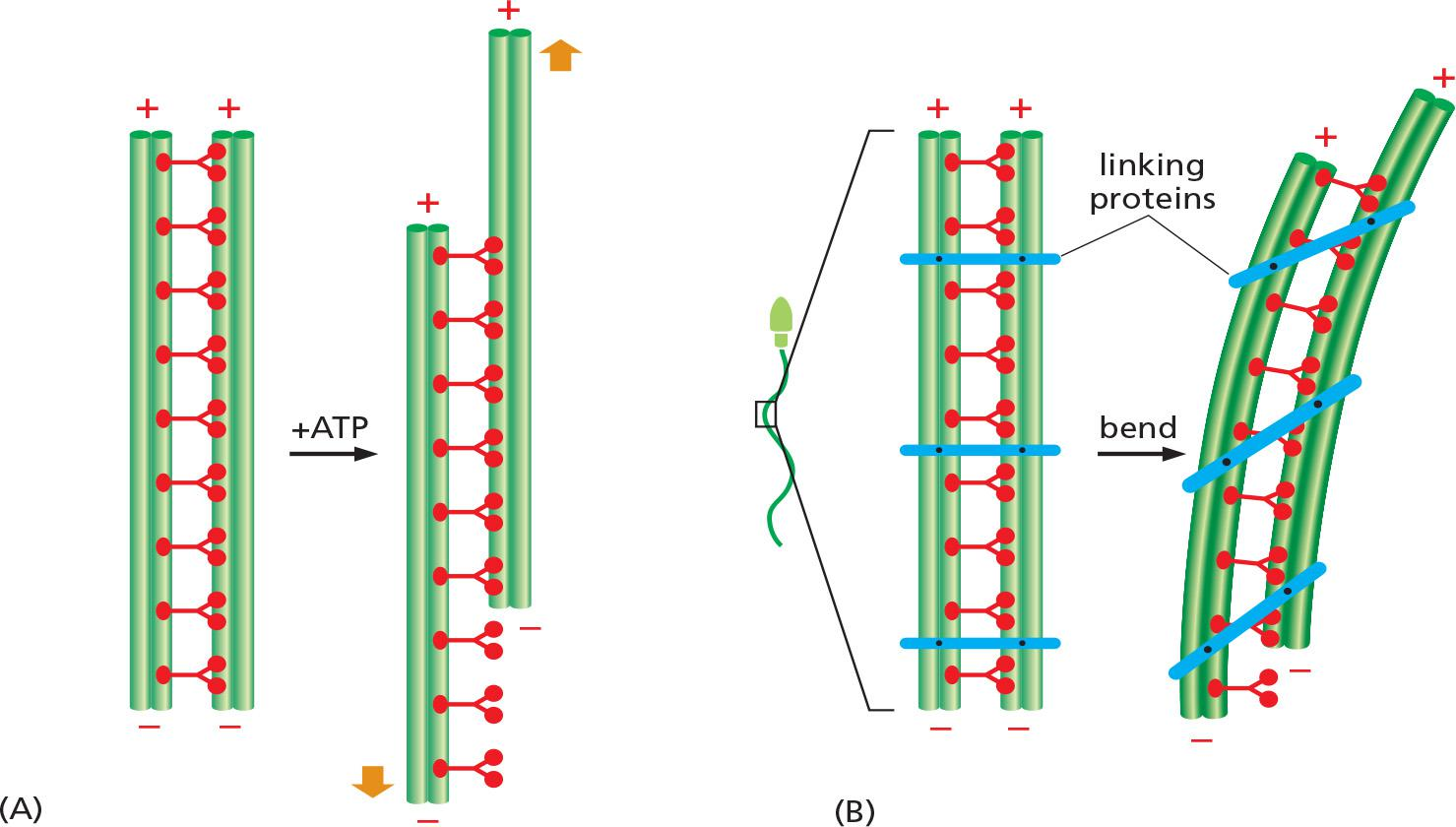
- The bending of axoneme due to fixed links between microtubule doublets
- The tail of dynein binds tightly to an A microtubule, while the large globular heads have an ATP-dependent binding site for a B microtubule.
- When the dynein heads hydrolyze their bound ATP, they move toward the minus end of the B microtubule, thereby producing a sliding force between the adjacent microtubule doublets in a cilium or flagellum
Primary cilia
Primary cilia are found on the surface of almost all cell types, where they sense and respond to the exterior environment, functions best understood in the context of smell and sight
Many cells possess a shorter, nonmotile counterpart of cilia and flagella called the primary cilium
Primary cilia can be viewed as specialized cellular compartments or organelles that perform a wide range of cellular functions, but share many structural features with motile cilia.
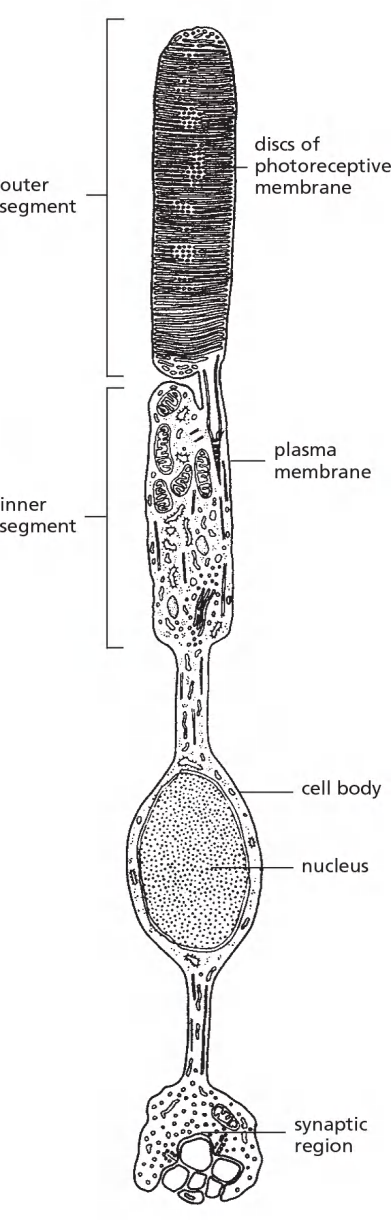
Genetic defects in dynein and kinesin linked to severe diseases
Kartagener’s syndrome (genetic defect in dynein)
- defective movement of cilia, leading to recurrent chest infections, ear/nose/throat symptoms, and infertility.
- Male sterility
- High susceptibility to lung infection
- Defects in left-right axis determination in development.
Charcot-Marie-Tooth disease (point mutation in a kinesin)
- point mutation in a kinesin family member that transports synaptic vesicle precursors down the axon
- The neuropathy of CMT affects both motor and sensory nerves.
- Motor nerves cause muscles to contract and control voluntary muscle activity such as speaking, walking, breathing, and swallowing.
- A typical feature: weakness of the foot and lower leg muscles, which may result in foot drop and a high-stepped gait with frequent tripping or falls.
- Foot deformities, such as high arches and hammertoes (a condition in which the middle joint of a toe bends upwards
五、Vesicle/organelle transport
Non-muscle myosin (e.g. myosin V) powered vesicle/organelle transport
Actin and myosin perform a lot of functions in non-muscle cells, usually towards the “+” end, only myosin VI moves to the “-” end
Myosin V motors carry a wide range of cargoes including mRNA, ER, and secretory vesicles along the actin cables.
Myosin V mediates the partitioning of organelles such as peroxisomes and mitochondria between mother and daughter cells
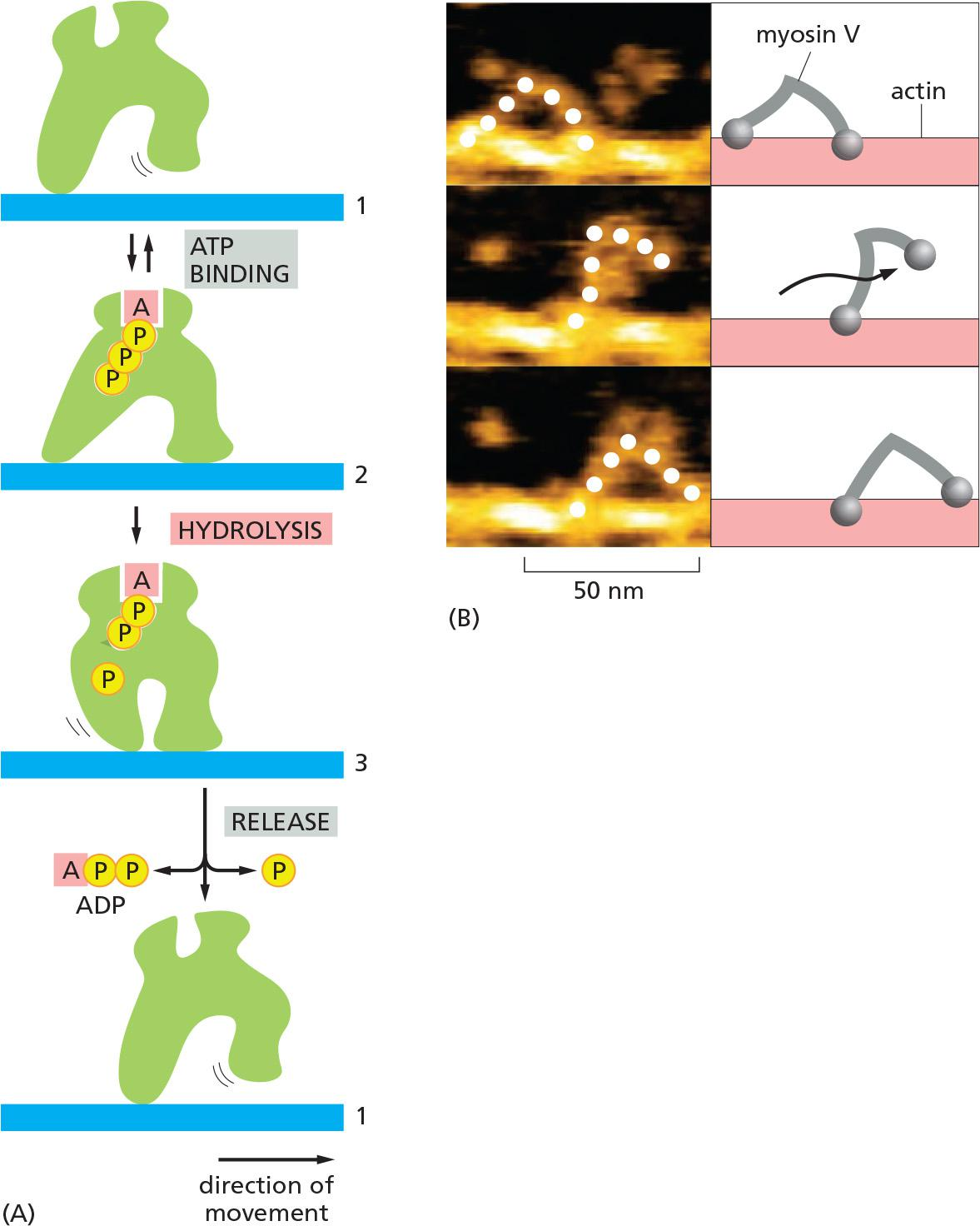
Regulated melanosome movements in fish pigment cells
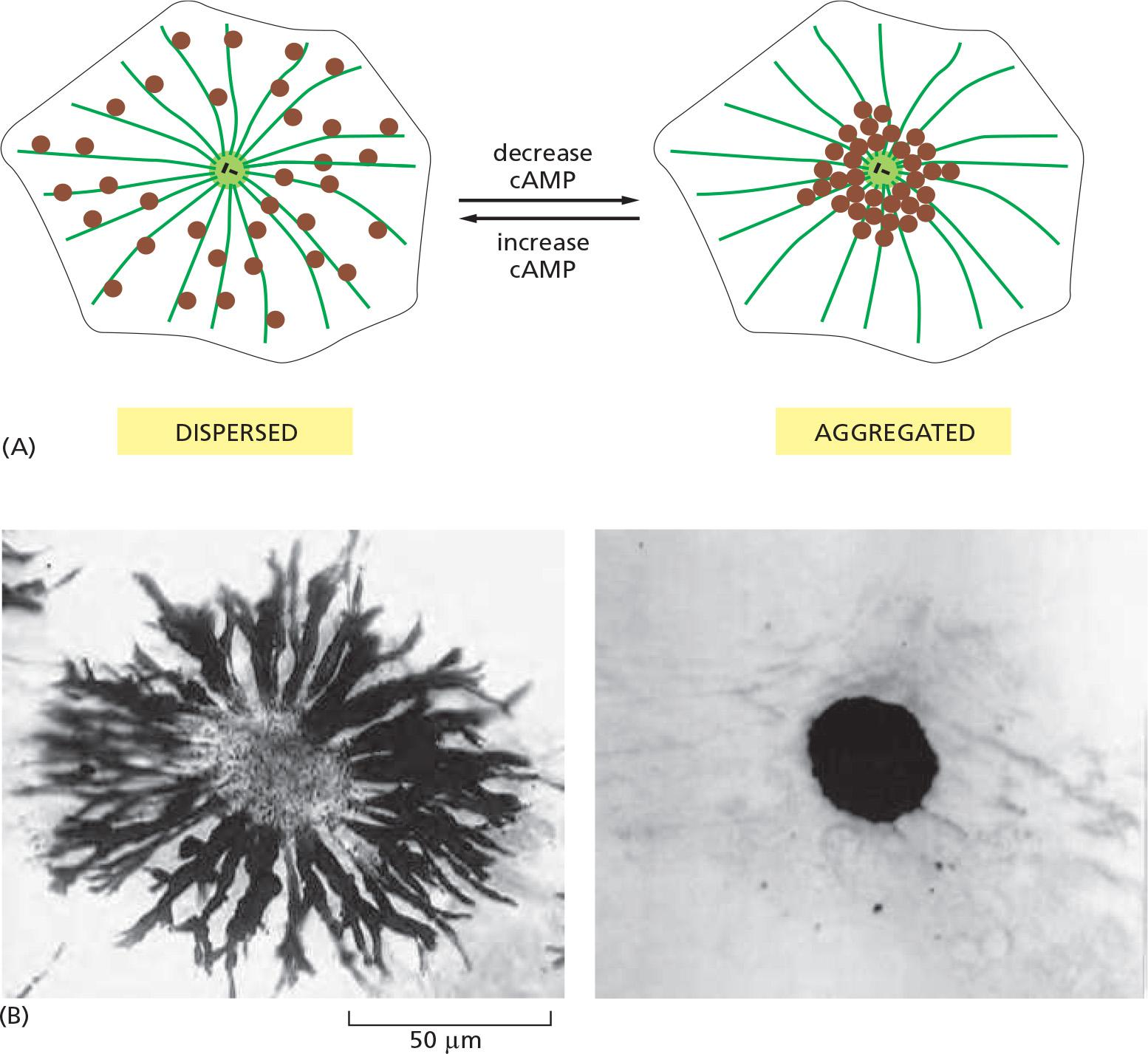
- It itself can’t transport cargo directly, and needs dynactin to link to cargo to be transported.